My work
Trying to provide light on the physiological basis of evolutionary ecology...
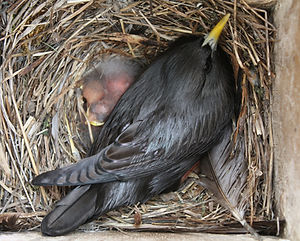
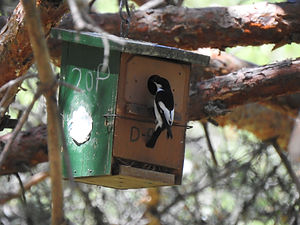

Maternal Effects
Phenotypic plasticity and eco-evolutionary dynamics
Avian immunity and pathogens
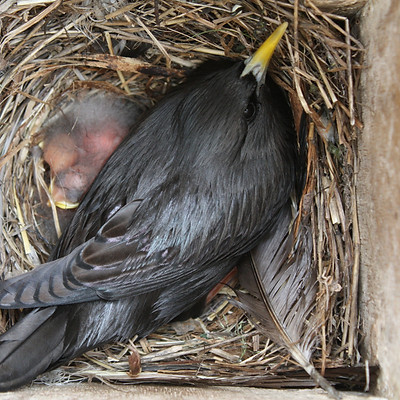
Maternal Effects
Currently, there is strong evidence that mothers can adjust the development and phenotype of their offspring based on environmental conditions. These adjusting mechanisms, named “maternal effects”, consist in an epigenetic modification on the offspring phenotype that results in an increase of transgenerational plasticity, ultimately affecting individual fitness (Mousseau and Fox 1998).
In the context of evolutionary ecology, there is increasing interest in the mechanisms behind these maternal effects, and specifically, in the mediating role of steroid hormones (reviewed in Groothuis et al. 2005; Groothuis and Schwabl 2008; Gil 2008). Steroid hormones are great candidates for studying these adaptive changes, as they regulate key developmental processes and also mediate adult responses to environmental changes (Groothuis and Schwabl 2008). Birds, and in our particular case the Spotless starling (Sturnus unicolor), are excellent models to study maternal effects because their embryos develop outside the mother's body in a sealed environment. This facilitates sample collection for descriptive studies and performing experimental manipulations of maternal effects by modifying yolk composition (Groothuis et al. 2005).
Our studies deep into the causes and consequences of the phenotypic plasticity caused by the variation in the levels of androgens in the egg with the aim of understanding how these processes mediate individual responses to life-history trade-offs (Groothuis et al. 2005; Gil 2008; Williams 2012). For the moment, the main results we have obtained during my PhD have allowed us to disentangle the separate and combined effects of the main steroids in the egg yolks of starlings (testosterone and androstenedione, Muriel et al. 2013). Also, we have discovered the existence of complex dose-response effects of these hormones on several traits of nestlings (Muriel et al. 2015a), and how the androgen effects vary according to the environmental context (Muriel et al. 2015b). In addition, we have observed that the cost of androgen levels, often emerge as sex-specific immunosuppression, as high hormone levels negative impact some components of the innate and adaptive axes of the immune system (e.g. lysozyme activity of the plasma and cell-mediated immunoresponsiveness) in male nestlings but not in females (Muriel et al. 2017). In the near future, we will explore the effects of these hormones on adult phenotype and several life-history traits in this model species.
References
Gil D. (2008). Hormones in avian eggs: physiology, ecology and behavior. Adv Study Behav. 38, 337–398.
Groothuis, T. G. G., Müller, W., von Engelhardt, N., Carere, C., and Eising, C. (2005). Maternal hormones as a tool to adjust offspring phenotype in avian species. Neurosci. Biobehav. Rev. 29, 329–352.
Groothuis TGG, Schwabl H. (2008). Hormone-mediated maternal effect in birds: mechanisms matter but what do we know of them? Philos Trans R Soc Lond B Biol Sci. 363:1647–1661.
Mousseau, T. A., and Fox, C.W. (1998). ‘‘Maternal Effects as Adaptations. ’’ Oxford University Press, New York, NY.
Muriel J, Pérez-Rodríguez L, Puerta M, Gil D (2013) Differential effects of yolk testosterone and androstenedione in embryo development and nestling growth in the spotless starling (Sturnus unicolor). Gen. Comp. Endocrinol. 194: 175-182.
Muriel J., Pérez-Rodríguez L., Puerta M., Gil D. (2015a). Diverse dose-response effects of yolk androgens on embryo development and nestling growth in a wild passerine. J. Exp. Biol. 218: 2241-2249.
Muriel J., Salmon P., Nunez-Buiza A., de Salas F., Pérez-Rodríguez L., Puerta M., Gil D. (2015b). Context-dependent effects of yolk androgens on nestling growth and immune function in a multibrooded passerine. J. Evol. Biol. 28: 1476-1488.
Muriel J., Pérez-Rodríguez L., Ortiz-Santaliestra M.E., Puerta M., Gil D. (2017). Sex-specific effects of high yolk androgen levels on constitutive and cell-mediated immune responses in nestlings of an altricial passerine. Physiol. Biochem. Zool. 90(1): 106-117.
Williams TD (2012) Hormones, life-history, and phenotypic variation: Opportunities in evolutionary avian endocrinology. Gen. Comp. Endocrinol, 176(3): 286-295.
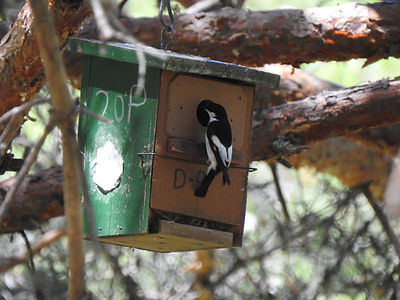
Phenotypic plasticity and eco-evolutionary dynamics
Birds, like other vertebrate species, need to adjust to environmental variations, and life-history traits should evolve towards maximizing fitness in the face of environmental variability and physiological trade-offs. Under changing environments, the evolution of life-history traits by natural selection will depend on genetically determined phenotypic diversity where selection can act upon to produce adaptive variations (Martínez-Padilla et al. 2017; Siepielski e al. 2017). The causes and consequences of variation in phenotype could play a leading role in the understanding of the evolutionary change of life-history traits. In this sense, evolutionary biology highlights a key mechanism: phenotypic plasticity, which is defined as the capacity of a single genotype to exhibit a range of phenotypes in response to variation in the environment (Fordyce 2006). Any biochemical, physiological, behavioral, and life history trait could show phenotypic plasticity (reviewed in Whitman and Agrawal 2009). Changes in these traits might be vital to survive and reproduce above maintenance levels and, hence, are necessary for the persistence of the population in heterogeneous and variable conditions (Pigliucci et al. 2006; Zunzunegui et al. 2011). Since plasticity was incorporated into the evolutionary framework, the environment has been recognized not only as playing a basic role in evolution by selecting among genetically fixed phenotypic variation but also as a force-generating that variation (Gilbert and Epel 2009; Pfennig et al. 2010). In summary, phenotypic plasticity could be considered as a key mechanism in buffering natural selection, producing new trait combinations, and shaping ecological speciation (Thibert-Plante and Hendry 2011).
We use the pied flycatcher (Ficedula hypoleuca) as a model species when investigating the role of environmental conditions to understand its consequences in ecological and evolutionary times, both on a temporal and geographical scale. The study area consists of a two-patch system comprising a mature oak forest of 9.3 ha near La Hiruela (Madrid) and a pine plantation of 4.8 ha near El Cardoso (Castilla La Mancha). Many aspects of the ecology of pied flycatchers have been studied in the study area over the past 37 years (e.g. Potti and Montalvo 1990; Potti et al. 2013; Camacho et al. 2016; 2019). Birds breeding in the oak and the pine forest differ in key life history and morphological traits, such as laying date (pine > oak), clutch size (oak > pine), and body size and weight (oak > pine), and there are also important differences in reproductive performance, measured as the number of hatchlings, fledglings, and recruits (pine > oak) (Camacho 2018). The foundation of a new pied flycatcher population next to the ancestral habitat of the species offered us a rare opportunity to document the emergence and change over time of a link between body size and dispersal propensity in the wild. Thus, previous data have shown that there is a sex-specific link between body size (tarsus length) and dispersal propensity in the pied flycatcher, and further show that this link emerged immediately after colonization of a non-natural habitat (Camacho et al. 2019). Currently, we are investigating the role of different environmental conditions on the evolutionary potential of secondary sexual traits, as well as quantifying their effect on the relative contribution of gene flow on the evolutionary dynamics of these traits.
References
Camacho, C., Canal, D., Potti, J. (2016). Natal habitat imprinting counteracts the diversifying effects of phenotype-dependent dispersal in a spatially structured population. BMC Evol. Biol. 16:158.
Camacho, C. 2018. Fine-scale population differentiation: ecological and evolutionary mechanisms involved. Ph.D. Dissertation. Universidad de Sevilla, Spain.
Camacho, C., Martínez-Padilla, J., Canal, D., & Potti, J. (2019). Long-term dynamics of phenotype-depended dispersal within a wild bird population. Behavioral Ecology, 30, 548–556.
Fordyce, J.A. (2006). The evolutionary consequences of ecological interactions mediated through phenotypic plasticity. J. Exp. Biol. 209(12): 2377-2383.
Gilbert S.F. and Epel D. (2009). Ecological developmental biology. Integrating epigenetics, medicine and evolution. Sunderland, MA: Sinauer Associates, Inc. 480 p
Martinez-Padilla, J., Estrada, A., Early, R. & Garcia-Gonzalez, F. (2017) Evolvability meets biogeography: evolutionary potential decreases at high and low environmental favourability. Proceedings of the Royal Society B: Biological Sciences 284 (1856), 20170516.
Pfennig D.W., Wund M.A., Snell-Rood E.C., Cruickshank T., Schlichting C.D. and Moczek A.P. (2010). Phenotypic plasticity's impacts on diversification and speciation. Trends Ecol. Evol. 25: 459-467.
Pigliucci, M., Murren, C.J. and Schlichting, C.D. (2006). Phenotypic plasticity and evolution by genetic assimilation. J. Exp. Biol. 209: 2362-2367.
Potti, J., Montalvo, S. 1990. Ocupación de áreas con nidales por el papamoscas cerrojillo (Ficedula hypoleuca). Ardeola 37:75-84.
Potti, J., Canal, D., Serrano, D. 2013. Lifetime fitness and age‐related female ornament signalling: evidence for survival and fecundity selection in the pied flycatcher. J. Evol. Biol. 26:1445−1457.
Siepielski, A. M., Morrissey, M. B., Buoro, M., Carlson, S. M., Caruso, C. M., Clegg, S. M., … Hereford, J. (2017) Precipitation drives global variation in natural selection. Science, 355, 959–962.
Thibert-Plante, X. and Hendry, A.P. (2011). The consequences of phenotypic plasticity for ecological speciation. J. Evol. Biol. 24: 326-342.
Whitman, D.W. and Agrawal, A.A. (2009). What is phenotypic plasticity and why is it important? In Phenotypic Plasticity of Insects: Mechanisms and Consequences (ed. D.W. Whitman and T.N. Ananthakrishnan), pp. 1-63. Enfield: Scientific Publishers.
Zunzunegui, M., Barradas, M.C.D., Ain-Lhout, F., Alvarez-Cansino, L., Esquivias, M.P. and Novo, F.G. (2011). Seasonal physiological plasticity and recovery capacity after summer stress in Mediterranean scrub communities. Plant Ecol. 212(1): 127-142.
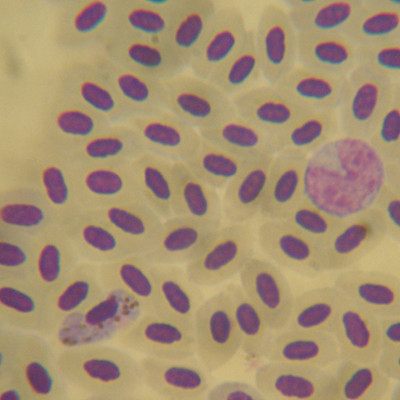
Avian immunity and pathogens
Parasitism is one of the main selective forces underlying the evolution of species, as this will have direct consequences on the host reproduction or survival (Sheldon and Verhulst 1996). The Red Queen hypothesis proposes that hosts and parasites are involved in a non-stop coevolutionary arms race, in which host resistance and parasite infectivity are under intense reciprocal selection (Jaenike 1978). However, the overall result of parasite-host interactions could be assessed through resistance or tolerance expressed by hosts and parasite infection success (Ebert 1994), which in turn affect parasite transmission rate and virulence (Bull 1994). In the case of avian malaria, these antagonistic interactions will drive the coevolution between birds and haemosporidian parasites. Hosts choose resistance strategies when trying to limit the infection intensity, whereas tolerance strategies will be selected when they need to reduce the costs derived from the activation of an immune response that could reduce its fitness (Råberg et al. 2007; Sorci 2013). In order to understand the ecological and evolutionary dynamics of these interactions, we are working to recognize the effects of these parasites on those physiological or immunological traits that directly affect the host fitness at an individual level.
In the case of chronic malaria infections, hosts could maintain low infection intensities through mechanisms involved in both the innate and acquired immune responses (Ots and Hõrak 1998; Ellis et al. 2014). White blood cells form the basis of the immune system, and their main function is to protect against pathogens. On the one hand, leukocytes associated with the innate immune system play a key role in the initial nonspecific protection mechanism during the early stages of infection (Schat et al. 2014). Thus, monocytes and granulocytes (heterophiles, basophils, and eosinophils) could provide an important measure of nonspecific immune function and host health status (Masello et al. 2009). On the other hand, the acquired immune system is highly specific and acts more effectively against parasites (Masello et al. 2009; Kaiser 2010). This adaptive immune system would imply a higher metabolic cost due to the immune memory processes that allow a rapid and specific defense against subsequent exposures to the same pathogen (Schat et al., 2014). When evaluating the immune response against parasites, various host factors must be taken into account that could be conditioning the interpretation of our results, such as age, sex or environmental conditions (Muriel et al. 2021a; Martin et al. 2008; Alkie et al. 2019). Currently, some of our ongoing work tries to delve into these factors, as well as the host's mechanisms to tolerate or cope with a parasitic infection.
Avian malaria and related haemosporidians (genera Haemoproteus, Plasmodium, and Leucocytozoon) are diverse and abundant protozoan parasites, with more than 4,100 parasite lineages nearly 2,000 species representing most bird clades (MALAVI database version 2.4.7, July 2021, Bensch et al. 2009). Predicting and mapping the distribution, abundance, and diversity of species is fundamental for resource management and biodiversity conservation planning (Franklin 2009). This is particularly important in the current global change scenario, where processes such as climate change and land-use changes are globally impairing wildlife populations (Newbold 2018). Because host–parasite interactions represent a selective pressure maintaining genetic variability in host populations, characterizing the structure of parasite communities is crucial to understanding ecosystem diversity and functioning (Ellis et al. 2020). For this reason, many of our last studies have focused on the variation on prevalence and lineage distribution of avian malaria in poorly studied biogeographic regions (Muriel et al. 2021b; García-Longoria et al. 2021), as well as those host families that have received less attention (Muriel et al. 2018). In fact, the AEET (Spanish Association of Terrestrial Ecology) recently awarded me a project to study the diversity, prevalence, and effects of malaria in the red-necked nightjars (Caprimulgus ruficollis), one of the most enigmatic and unknown species of the Western Palearctic (Sáez-Gómez et al. 2015). These general studies in non-model species are essential in the ecology of disease transmission for the understanding of the role of migratory species as a reservoir of diseases and in the translocation and dispersal of pathogens between geographically distant areas.
References
Alkie, T.N., Yitbarek, A., Hodgins, D.C., Kulkarni, R.R., Taha-Abdelaziz, K., Sharif, S. (2019). Development of innate immunity in chicken embryos and newly hatched chicks: a disease control perspective. Avian Pathology 48: 288-310
Bensch, S.; Hellgren, O.; Pérez-Tris, J. (2009). MalAvi: A public database of malaria parasites and related haemosporidians in avian hostsbased on mitochondrial cytochrome b lineages.Mol. Ecol. Resour. 9, 1353-1358
Bull, J.J. (1994). Virulence. Evolution, 48, 1423–1437.
Ebert, D. (1994). Virulence and local adaptation of a horizontally transmitted parasite. Science, 265, 1084–1086.
Ellis, V.A., Kunkel, M.R., Ricklefs, R.E. (2014). The ecology of host immune responses to chronic avian haemosporidian infection. Oecologia 176: 729-737.
Ellis, V.A.; Huang, X.; et al. (2020). Explaining prevalence, diversity and host specificity in a community of avian haemosporidian parasites. Oikos, 129, 1314-1329
Franklin, J. (2009) Mapping Species Distributions: Spatial inference and Prediction; Cambridge University Press: Cambridge, UK
García-Longoria L., Muriel J., et al. (2021). Diversity and host assemblage of avian haemosporidians in different terrestrial ecoregions of Peru. Current Zoology, zoab030
Jaenike, J. (1978). A hypothesis to account for the maintenance of sex within populations. Evol. Theor. 3, 191-194.
Kaiser, P. (2010). Advances in avian immunology-prospects for disease control: A review. Avian Pathology 39: 309-324.
Martin, L.B., Weil, Z.M., Nelson, R.J. (2008). Seasonal changes in vertebrate immune activity: Mediation by physiological trade-offs. Philos. Trans. R. Soc. B: Biol. Sci. 363: 321-339.
Masello, J.F., Choconi, R.G., et al. (2009). Do leucocytes reflect condition in nestling burrowing parrots Cyanoliseus patagonus in the wild? Comp. Biochem. Physiol. A Mol. Integr. Physiol. 152: 176-181.
Muriel, J., Graves, J. A., Gil, D., Magallanes, S., Salaberria, C., Casal-López, M., Marzal, A. (2018). Molecular characterization of avian malaria in the spotless starling (Sturnus unicolor). Parasitol. Res., 117(3), 919–928.
Muriel, J., Vida, C., Gil, D., Pérez-Rodríguez, L. (2021a). Ontogeny of leukocyte profiles in a wild altricial passerine. J. Comp. Physiol. B. 191:195–206.
Muriel, J., Marzal, A., et al. (2021b). Prevalence and diversity of avian haemosporidians may vary with anthropogenic disturbance in tropical habitats in Myanmar. Diversity, 13, 111.
Newbold, T. (2018) Future effects of climate and land-use change on terrestrial vertebrate community diversity under different scenarios. Proc. R. Soc. B, 285, 20180792
Ots, I., Hõrak, P. (1998). Health impact of blood parasite in breeding great tits. Oecologia 116: 441-448.
Råberg, L., Sim, D., Read, A.F. (2007). Disentangling genetic variation for resistance and tolerance to infectious diseases in animals. Science 318: 812-814.
Sáez-Gómez, P., et al. (2015) El chotacabras cuellirrojo en Doñana: un elemento conciliador entre agricultura y conservación. Quercus 355, 25-32.
Schat, K.A., Kaspers, B., Kaiser, P. (2014) Avian Immunology, 2nd ed.; Academic Press: San Diego, CA, USA.
Sheldon, B. C.; Verhulst, S. (1996). Ecological immunology: costly parasite defenses and trade-offs in evolutionary ecology. Trends Ecol. Evol. 11: 317-321.
Sorci, G. (2013). Immunity, resistance and tolerance in bird-parasite interactions. Parasite Immunology 35: 350-361.